Accreting Neutron Stars
Neutron stars are compact remnants of massive stars with masses of about 1.4 M☉ and radii of about 10 km. In contrast to black holes, they have a solid surface. Some neutron stars have magnetic fields of the order of 1012 Gauss, the strongest magnetic fields known in the universe. If matter from a companion star in a binary system is accreted onto the neutron star, its potential energy is released in radiation. This process makes accreting neutron stars bright X-ray sources. The radiation processes that produce the X-ray radiation are governed by the strong magnetic field of the NS and also by the mass accretion rate, i.e., the amount of matter that falls onto the neutron star.
Due to strong magnetic field of the neutron star, ionized matter is directed onto the magnetic poles and funneled into accretion columns, where the matter is finally decelerated from its free-fall velocity of 100000 km/s and more until it settles on the neutron star surface. This accretion column is the region where most of the X-ray radiation is produced. As the neutron star rotates, X-ray pulsations are observed if the rotational axis is misaligned with the magnetic field axis.
Modelling accretion columns
In collaboration with scientists at the Navy Research Laboratory, the George Mason University, NASA’s Goddard Space Flight Center, and Moscow State University we are developing a comprehensive, physically motivated model of the accretion column which includes the proper treatment of radiative transfer in the presence of strong magnetic fields for a wide range of mass accretion rates. The physical radiation processes contributing mostly to the X-ray spectral formation are mostly Comptonization, bremsstrahlung, and black body radiation. The interaction of the radiation with matter can be assessed analytically and numerically in different mass accretion regimes and for different underlying assumptions.
The most characteristic spectral features in the X-ray spectra of accreting NS are Cyclotron Resonant Scattering Features (CRSF) or cyclotron lines. They result from the resonant scattering of photons with electrons whose momenta parallel to the magnetic field are quantized into discrete energy levels. We are developing a Monte-Carlo code to simulate the line shapes, scattering cross sections, and mean free paths for various configurations of the accreting medium and incident radiation. Comparison of these simulations with observed cyclotron lines will allow us to infer the physical conditions in the accretion columns, e.g, the plasma temperature or its optical depth.
Finally, the compactness of the NS affects how light travels from its origin to a distant observer since photon trajectories cannot be approximated by straight lines any more in the realm of strong gravity. This effect can be observed in complex and asymmetric pulse profiles, i.e. the flux variation over one rotational period. Our group is working on a simulation code for pulse profiles that calculates the expected pulse profile for arbitrary configurations of accretion columns in a general relativistic framework. These can be compared to observational data and are the basis for an advanced investigation of the emission geometry of accretion columns.
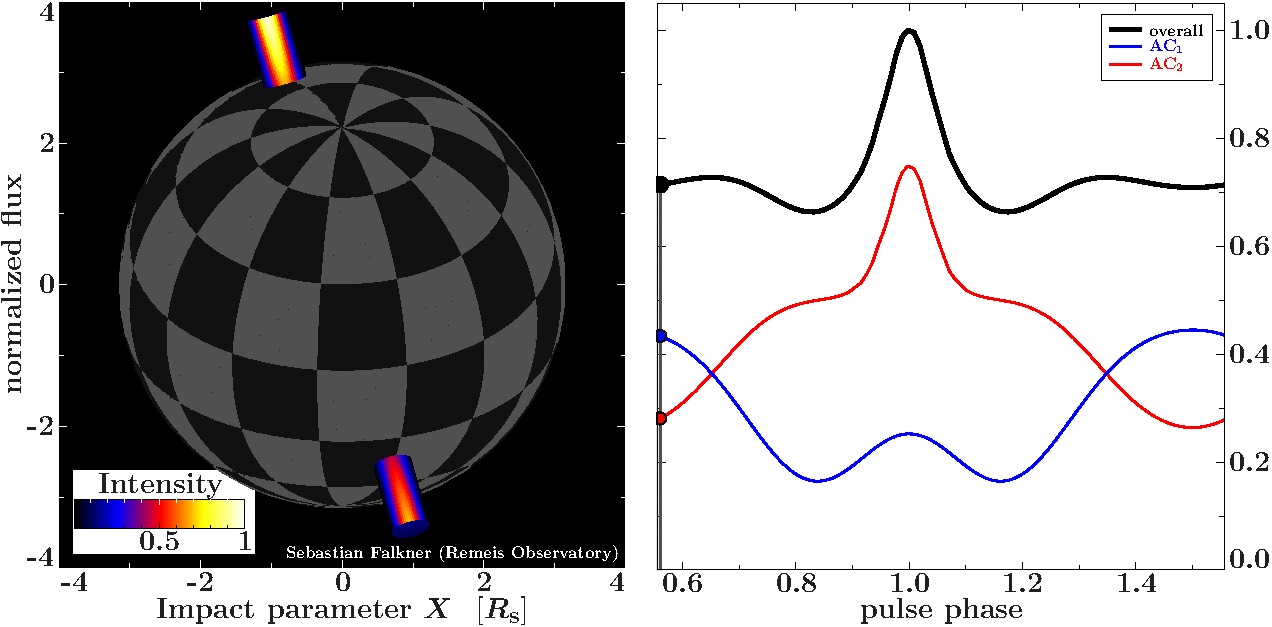
Observing the spectral evolution
We are using large amounts of observational data of previous and current X-ray missions (e.g., RXTE, INTEGRAl, Suzaku, and NuSTAR) to conduct phenomenological studies of accreting NS. Due to their simplicity, phenomenological models are more suitable to be applied to a larger sample of observations and are generally used to investigate the spectral evolution of individual sources over time or X-ray flux or to compare the behavior of different sources.
Using phenomenological models, we were able to detect transitions between different accretion regimes which have been proposed by theory or map the cyclotron line forming region by tracking the line parameters at different luminosity levels. While the ultimate goal still is to develop a self-consistent model of accreting NS, the current observations and phenomenological analyses provide crucial feedback for theory.
Binary orbit determination
For a complete picture of these systems, studies of neutron stars have to include interaction of the neutron star with its companion and the mass transfer in the binary system need to be understood. For most cases, the evolution of the neutron star’s pulse period can be determined at very high precision and pulsar timing techniques provide very sensitive tools for investigations of the binary system. Changes in the pulse period can either be intrinsic, i.e., due to angular momentum transfer from the companion onto the neutron star or the pulse period changes can be caused by a Doppler shift due to the orbital motion of the neutron star. In combination with flux measurements, the first contribution allows us to determine the angular momentum that is carried by the accreted material. This is an indicator for its origin (e.g., an accretion disk or a stellar wind). The second contribution allows us to derive the orbital parameters, such as the eccentricity or the projected axes of the orbit.